Can a single amino acid mutation change the course of the COVID-19 pandemic?
Viruses mutate in the course of an epidemic as a result of selective pressure or genetic drift. Resulting mutations may result in enhanced fitness or better efficiencies in evading the host’s immune system. Despite the low mutation rates observed in coronaviruses, SARS-CoV-2 has already accumulated a vast sequence diversity since the beginning of the pandemic, suggesting it is still adapting to its new human host. How will these changes influence treatments and vaccines currently in clinical trials?
How fast is SARS-CoV-2 mutating?
Data availability has been crucial to design and repurpose treatments for the new COVID-19 disease as well as to understand the course of the COVID-19 disease. Since the publication of the first whole-genome sequence of SARS-CoV-2 in early January, more than 67,700 sequences of the new strain of SARS-CoV-2 have been submitted to a publicly available database curated by the Global Initiative on Sharing All Influenza Data (GISAID). GISAID has since become a crucial resource for scientists promoting rapid data sharing among different research groups around the world.
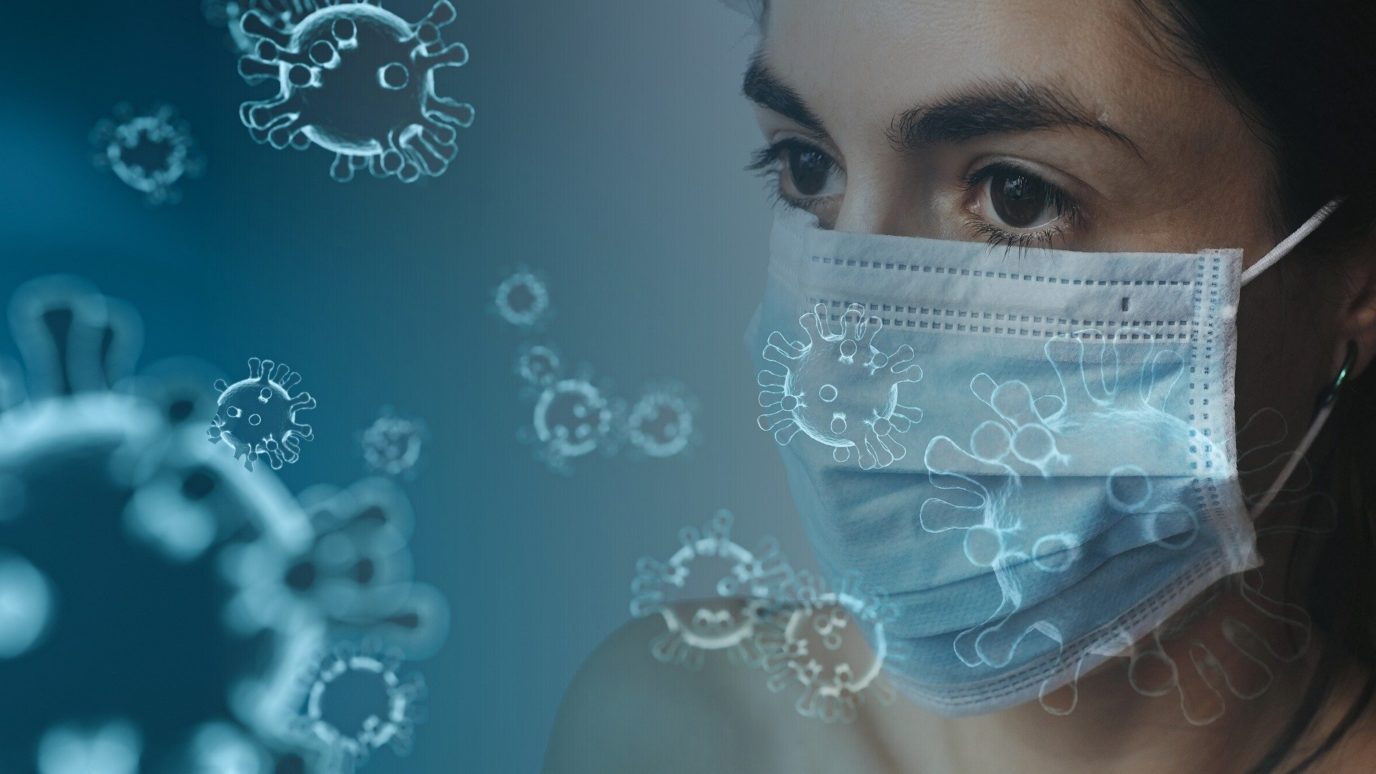
This invaluable resource allowed crucial insights into the dissemination and mutation rates of the new zoonotic strain of coronavirus. In addition to allowing the identification of conserved domains (better suited for drug and vaccine development), this resource continues to help scientists detecting new variants of the virus, as well as correlate mutations with in vitro and in vivo infectivity.
Studies show that, in general, coronaviruses have lower sequence diversity and mutation rates in comparison to more pervasive viruses such as influenza and HIV. A behavior probably caused by the genetic proofreading mechanisms present in this family of viruses. Interestingly, despite this characteristic, SARS-CoV-2 has already accumulated significant genetic diversity since the beginning of the pandemic.
Experts explain that these changes may be the result of a natural selection process driven by the interaction between SARS-CoV-2 and the human immune system, and by recombination between different variants or strains of coronavirus.
Recurrent mutations found in the genome of SARS-CoV-2
A study published in early May identified almost 200 recurrent mutations (homoplasies) in the genome of SARS-CoV-2, nearly 80% of which representing non-synonymous changes at the protein level. Most of these mutations were observed in non-structural proteins Nsp6 (coronavirus replicase), Nsp11 (coronavirus guanine-N7 methyltransferase), and Nsp13 (zinc-binding domain), and in the spike glycoprotein.
In SARS-CoV-2, all non-structural proteins are encoded by the Orf1ab polyprotein which constitutes about two-thirds of its genome and undergoes an autoproteolytic process to form all 16 known non-structural proteins. Mutations in this region are consistent with previous studies with SARS-CoV-1 and MERS-CoV, where homoplasies were identified in proteins Nsp9 (SARS), Nsp13 (SARS), and Nsp6 (MERS).
Mutations in the Nsp6 protein observed both in SARS-CoV-2 and MERS-CoV, suggest this adaptation may be beneficial. In fact, Nsp6 is likely involved in autophagy restriction in CoVs and mutations may favor infection by evading the delivery of viral components to lysosomes for degradation.
More recently, researchers detected a more prevalent mutation with known effects in SARS-CoV-2 infectivity – the D614G amino acid substitution in the C-terminal region of the S1 domain of the spike protein. The mutation is caused by a single nucleotide change (A to G) at position 23,403 in the Wuhan reference strain. Detected in early March, the variant (termed “G clade” in GISAID) was shown to be frequently accompanied by three additional C to T mutations at positions 241, 3,037 (silent), and 14,408 (results in an amino acid change in the RNA-dependent RNA polymerase).
Shop these products
Has the D614G mutation changed SARS-CoV-2 infectivity?
The transition from the D614 to the G614 variant may have initially occurred in China and spread quickly through Europe, where the variant quickly became dominant and continued spreading across North America, Oceania, and finally Asia. The new variant, observed only in low frequency in March (26%), now accounts for almost 80% of all submitted SARS-CoV-2 samples.
This rapid increase in frequency suggests improved infectivity in comparison to the Wuhan original variant. An effect that has been confirmed in vitro using HEK cells. Phenotypically, the D614G variant is shown to have a greater number of functional spikes on its surface in comparison to the original variant. Additionally, the mutation was shown to stabilize the interaction between the S1 and S2 domains and limit S1 shedding, resulting in better overall infectivity.
The substitution presumably arose to compensate for the presence of the furin cleavage site (absent in other CoVs). But despite increasing the viral load in patients in comparison to the original variant, it is still unclear whether this mutation will impact disease severity.
What are the implications for therapy, research, and diagnostics?
It is still unclear whether the D614G mutation will be harder to control than the original Wuhan strain. So far no evidence exists on whether this mutation translates into increased disease severity in patients infected by the new variant. Nevertheless, since most treatments and vaccines in clinical trials target the spike protein of SARS-CoV-2, it remains a possibility that this change could potentially reduce the efficiency of candidate vaccines or render the virus resistant to specific treatments.
However, more studies are necessary to understand the role of this single mutation in the course of SARS-CoV-2 infections. Studies assessing the severity of point mutations can be done with variant-specific antibodies. For these projects, the ability to exert precise control over cross-reactivity and specificity is crucial. In this context, the use of phage display technology is markedly advantageous in comparison to other conventional processes. In fact, by using chemically synthesized peptides, it is possible to refine the specificity of a given antibody to a given protein form and thus precisely determine the abundance and evolution of different variants of the disease.
Unlike sequence-dependent methods, the use of fluorescent-labeled antibodies and fluorescence-activated cell sorting (FACS) allow phenotypic evaluation and real-time determination of different protein forms in patient samples. These antibodies can either serve as precise diagnostic tools to complement COVID-19 studies or eventually serve to understand the differences in the mechanism of infection between the original strain and the more recent “G clade” of SARS-CoV-2.
Concluding remarks
Coronaviruses mutate slower than other pervasive viral pathogens such as influenza. However, since the beginning of the pandemic, SARS-CoV-2 has already developed important changes. The most important and frequent mutations were found in the Nsp6 protein, responsible for restricting autophagy, and in the spike protein (D614G).
The implications of these mutations for SARS-CoV-2 fitness and infectivity are already well understood. Patients carrying this variant were shown to have significantly higher viral loads, which in turn contributes to a fast propagation of the disease. But the implications for disease severity or efficiency of current candidate vaccines and treatments are still unknown. For this reason, it is increasingly important to incorporate the use of precise diagnostic tools to assess the implications of these changes in the course of the disease. In this context, antibodies arise as the best reagents for research and diagnostics by allowing the distinction between mutated and native forms of functionally important proteins.
- Carmona-Gutierrez, D. et al. Digesting the crisis: autophagy and coronaviruses. Microb Cell. 2020; 7(5): 119–128. doi: 10.15698/mic2020.05.715
- Global Initiative on Sharing All Influenza Data (GISAID). hCoV-199 database. Available from: https://www.gisaid.org/
- Grubaugh, N.D. et al. Making sense of mutation: what D614G means for the COVID-19 pandemic remains unclear. Cell. 2020. doi: 10.1016/j.cell.2020.06.040
- Khailany, R. A. et al. Genomic characterization of a novel SARS-CoV-2. Gene Rep. 2020; 19: 100682. doi: 10.1016/j.genrep.2020.100682
- Korber, B. et al. Tracking changes in SARS-CoV-2 Spike: evidence that D614G increases infectivity of the COVID-19 virus. Cell. 2020. doi: 10.1016/j.cell.2020.06.043
- van Dorp, L. et al. Emergence of genomic diversity and recurrent mutations in SARS-CoV-2. Infect Genet Evol. 2020; 83:104351. doi: 10.1016/j.meegid.2020.104351
- Zhang, et al. The D614G mutation in the SARS-CoV-2 spike protein reduces S1 shedding and increases infectivity. bioRxiv. 2020. doi: 10.1101/2020.06.12.148726