Essential steps for the successful manufacturing of therapeutic antibodies
In a specific sense, antibody production steps include the synthesis and purification of the antigen (peptide, protein, or DNA), the selection of germlines or clones with high affinity to the intended target, and the recombinant or non-recombinant production of these biopharmaceuticals. Currently, recombinant antibody production methods offer a greater potential for scalability and they are the preferred approach used in therapeutic antibody generation. In this article, we will cover the different recombinant production processes, as well as present the current challenges and future perspectives.
Selection of the proper antibody production steps
Scientific progress and technological advances continue to revolutionize antibody production since the discovery of the hybridoma technology in 1975 and commercialization of the first therapeutic antibody in 1986.
Due to their high affinity and specificity towards other biological molecules, antibodies have been widely used to treat and diagnose a broad range of human diseases. For this reason, they have grown to represent the largest and fastest-growing class of biopharmaceutical molecules of our century.
Antibody production steps form a complex network of stages and decisions that need to be considered and tailored according to the expected outcomes of each project:
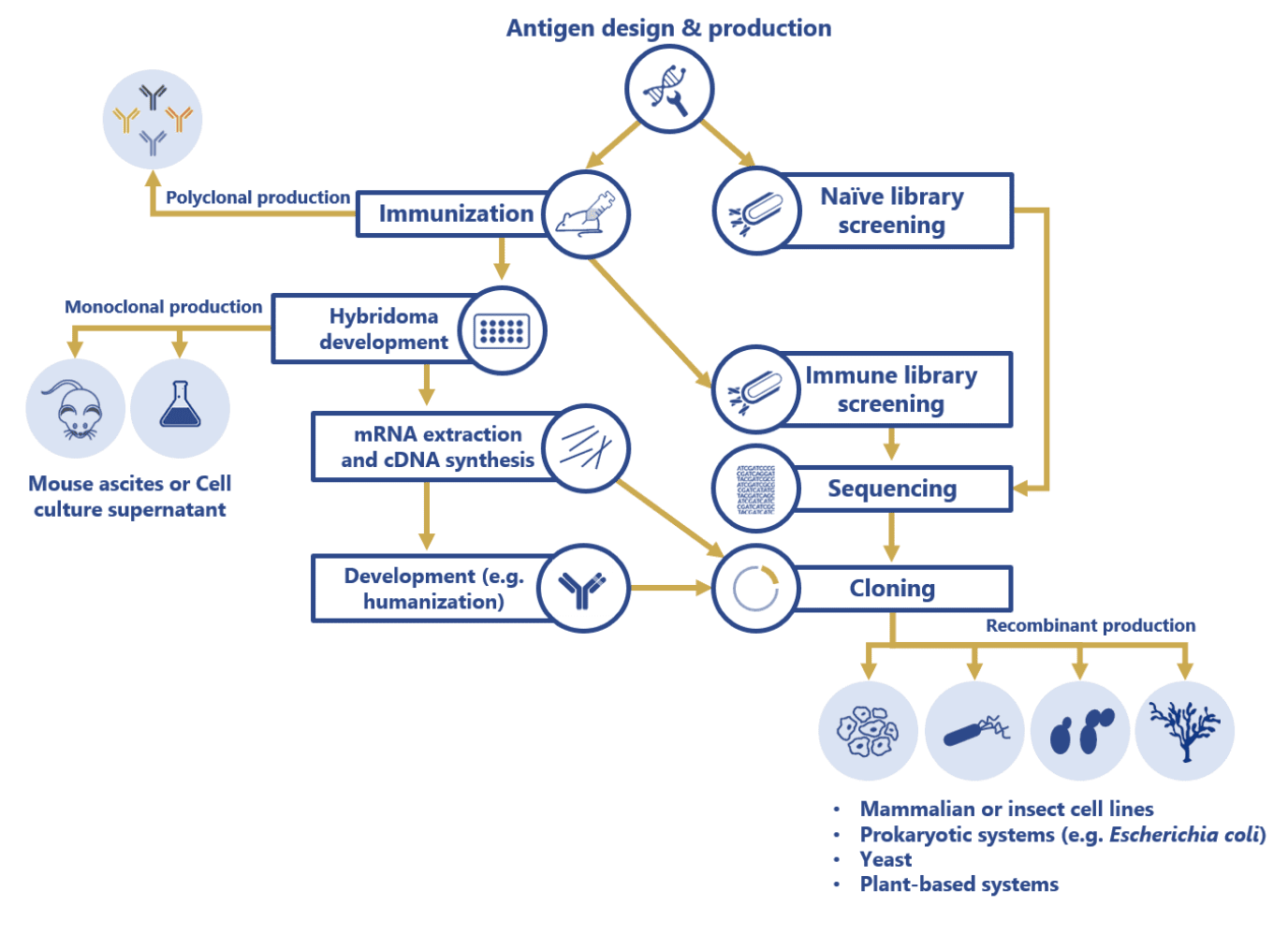
Currently, commercial therapeutic antibody production has shifted from the use of unstable hybridoma cell lines (ascites and cell culture supernatant production methods) to sequencing and cloning of the antibody sequences, and subsequent transfection into diverse, robust and more stable biological systems including mammalian, prokaryotic, yeast, insect and plant cell lines. Contrarily, antibodies intended for analytical and research purposes are usually produced using less time-consuming processes such as antiserum purification (polyclonal antibodies) or mouse ascites and cell culture supernatant methods (monoclonal antibodies).
But recombinant technologies continue to push the technical limits of the different antibody production steps by reducing the production and maintenance costs thanks to their ability to:
- Increase antibody production yields
- Decrease lead times from the generation to the large-scale production
- Broaden the functionality of these biopharmaceuticals (i.e. bispecific antibodies)
Antibody production steps in mammalian cell lines
The vast majority of commercialized antibodies belong to the IgG class and they consist of two heavy chains and two light chains linked by disulfide bonds and divided into a constant (Fc), variable (Fab) and antigen-binding (Fv) region. Most of these IgG antibodies undergo a complex post-translational modification process termed glycosylation in their Fc region.
And although this region does not directly interact with the antigen, it is important for the stabilization of the antibody structure, for the recruitment of immune effector cells or for eliminating the risk of immune responses in patients due to incompatible N-glycans.
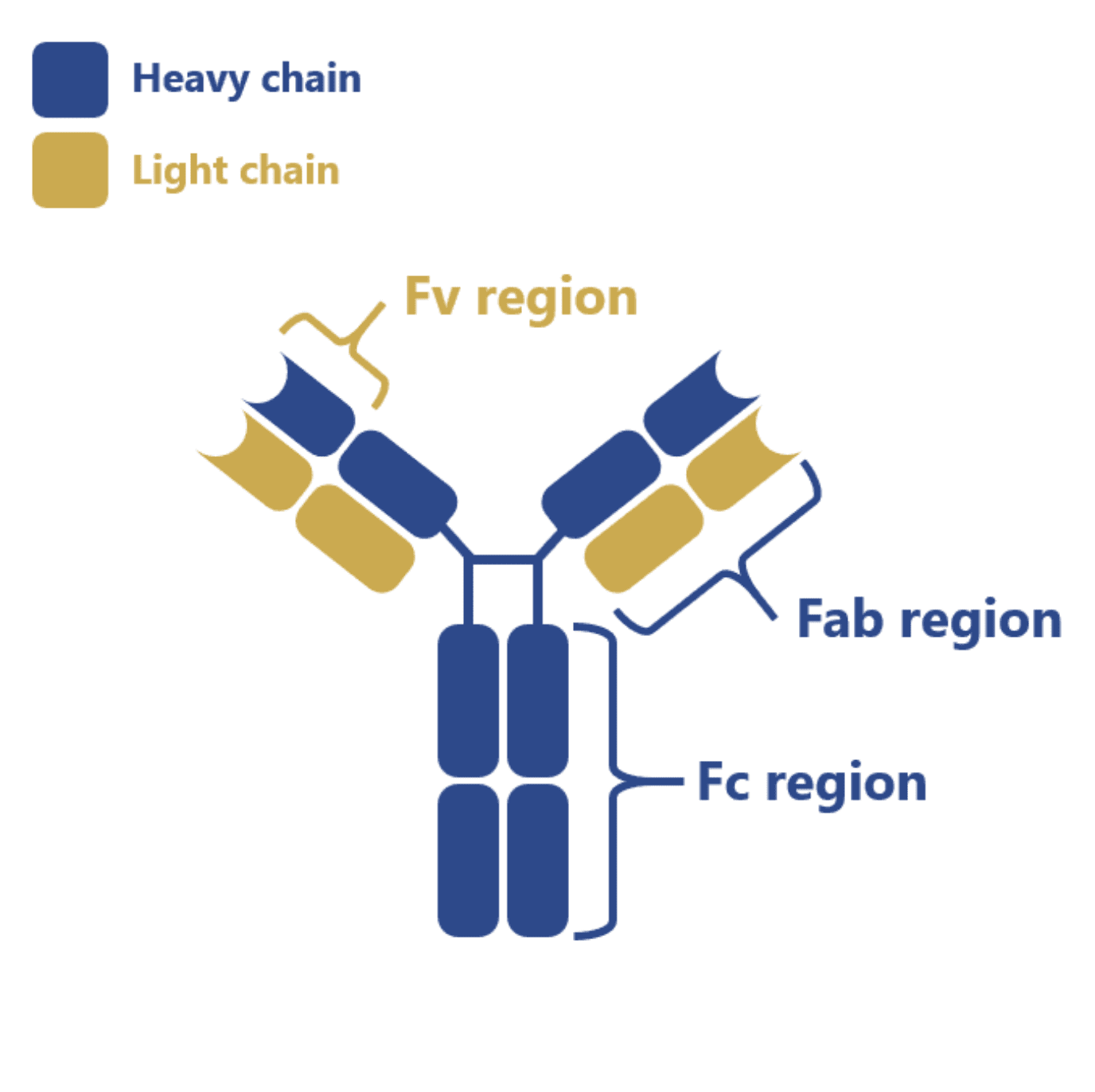
Most non-human expression systems either cannot perform post-translational glycosylation or they present very distinct glycosylation patterns when compared to human cell lines. That is why, historically, the use of mammalian cell lines able of human-like glycosylation has played a dominant role in antibody production.
Traditionally, Chinese hamster ovary (CHO) cell lines have been the preferred stable system for recombinant antibody production. The use of these cell lines presents several advantages including its relatively simple and human-like glycosylation process, fast growth rates and high antibody production titers, ease of genetic manipulation, good proliferation in large-scale suspension cultures and adaptability to protein-free medium.
Many antibodies approved for therapy have been obtained from recombinant production in CHO cells. However, the culture medium and conditions of these cell lines need to be carefully optimized to maximize and stabilize antibody production. For this reason, the generation and subsequent selection of stable CHO germlines is usually time-consuming, technically-demanding and, for this reason, it is reserved for large-scale commercial antibody production. While transient systems such as embryonic kidney (HEK 293), amniotic (CAP), a hybrid of HEK 293 and lymphoma (HKB-11), and embryonic retina (PER.C6) are preferably used for preliminary studies.
Despite the technological advances of the last decade, the use of mammalian systems, especially stable systems, still has a very high production cost. These cells require the use of expensive media and they are highly susceptible to viral and bacterial infections which may hamper long-term manufacturing processes. Moreover, mammalian systems are hard to scale-up, requiring long and incremental scale-up steps which generate increased processing times. For these reasons, researchers are increasingly interested in alternative antibody production methods.
Antibody production steps in microbial systems
Bacteria, such as Escherichia coli, and yeast systems, such as Saccharomyces cerevisiae and Pichia pastoris, are unable to perform human-like glycosylation. However, functional Fab and Fv regions suffice to bind and recognize antigens. Thus, microbial systems are increasingly gaining traction for the production of antibody fragments (e.g. single-chain antibody fragments – scFvs).
There are several advantages in using antibody fragments, namely, the decreased production cost (inexpensive culture media) and lead time, increased ease of genetic manipulation in comparison to mammalian systems and increased tissue penetration.
S. cerevisiae was the first microbial system to be employed in the production of recombinant biopharmaceuticals. Presently, this system is also well-established and the preferred method for the production of camelid’s heavy chain antibody fragments.
P. pastoris is also a commonly used microbial system that can be engineered to produce both full-length human-like glycosylated antibodies and antibody fragments. To date, at least two antibody fragments produced by this microbial system are already on the market: Anti-IL-6R Nanobody®, used to treat rheumatoid arthritis and Nanobody® ALX00171, used to treat respiratory syncytial virus (RSV) infections.
Due to the lack of glycosylation pathways in E. coli, the use of this Gram-negative microbial strain has been restricted to the production of non-glycosylated antibody fragments. But this limitation may soon be solved as researchers continue to extend the versatility and potential of these strains for N-glycosylation by genetic engineering.
Despite the lower production costs associated with these microbial systems, several drawbacks have been limiting their application. The fact that none of these systems can perform human-like glycosylation hinders their use for full-length antibody production. Moreover, recombinant antibodies produced by E. coli systems have a high risk of endotoxin contamination. Although these cells are easily cultured and genetically manipulated, for therapeutic applications it is necessary to perform additional purification steps to remove these endotoxins.
Antibody production steps in plants
After the production of the first plant-derived recombinant antibodies by two independent research groups in 1989, the use of plant-based systems (cell cultures and transgenic plants) continues to gain traction as an alternative approach for low-cost recombinant antibody production.
Plants and mammals share conserved secretory pathways which result in similar protein folding and post-translational modifications. For this reason, plant-derived recombinant antibodies are expected to have reduced immunogenicity, making them ideal for therapeutic applications.
There are many methods for transforming plants and allowing them to produce full-length IgG-like antibodies. However, presently, the most commonly used method is agrofiltration. This method achieves high expression levels by the transient transformation of plant systems using Agrobacterium tumefaciens-mediated gene transference. This process was first applied to tobacco leaf tissue and shown to facilitate the DNA transfer with high expression levels without the need to transform the entire plant.
The potential benefits of using plants for the manufacture of biopharmaceuticals include high scalability and rapid production, a low burden of human pathogens, and the ability to carry out human-like glycosylation of full-length antibodies. For these reasons, plants are increasingly becoming viable biofactories of recombinant protein production.
Concluding remarks
Presently, the production of full-length IgG-like antibodies (monoclonal, recombinant or bispecific) is predominantly obtained from stable or transient mammalian expression systems, such as Chinese hamster ovary (CHO). Contrarily, single-chain antibody fragments are preferably produced in E. coli due to the low-costs, fast-growth and high production rates of these prokaryotic cell lines.
However, as the demand for new therapeutic antibodies continues to increase, so does the need to develop more cost-effective systems. To overcome the drawbacks of traditional expression systems, the use of plant-based and microbial-based technologies continues to gain traction and they are expected to define commercial production of therapeutic antibodies in the next decades.
- Barta, A. et al. The expression of a nopaline synthase — human growth hormone chimaeric gene in transformed tobacco and sunflower callus tissue. Plant Mol Biol. 1986; 6(5):347-357. doi: 10.1007/bf00034942
- Buyel, J. F. et al. Very-large-scale production of antibodies in plants: The biologization of manufacturing. Biotechnol Adv. 2017;35(4):458-465. doi: 10.1016/j.biotechadv.2017.03.011
- Carvalho, L. S. et al. Production Processes for Monoclonal Antibodies. Fermentation Processes. 2017. doi: 10.5772/64263
- Fisher, A.C. et al. Production of secretory and extracellular N-linked glycoproteins in Escherichia coli. Appl. Environ. Microbiol. 2011; 77(3):871–881. doi: 10.1128/aem.01901-10
- Hiatt, A. et al. Production of antibodies in transgenic plants. Nature. 1989; 342(6245):76-78. doi: 10.1038/342076a0
- Leenaars, M. and Hendriksen, C. F. M. Critical Steps in the Production of Polyclonal and Monoclonal Antibodies: Evaluation and Recommendations. ILAR Journal. 2005 ; 46(3):269–279. doi : 10.1093/ilar.46.3.269
- Lizak, C. et al. N-Linked glycosylation of antibody fragments in Escherichia coli. Bioconjug. Chem. 2011; 22(3):488–496. doi: 10.1021/bc100511k
- Nandi, S. et al. Techno-economic analysis of a transient plant-based platform for monoclonal antibody production. mAbs. 2016;8(8):1456-1466. doi: 10.1080/19420862.2016.1227901
- Spadiut, O. et al. Microbials for the production of monoclonal antibodies and antibody fragments. Trends Biotechnol. 2014; 32(1): 54–60. doi: 10.1016/j.tibtech.2013.10.002