Antibody production: cells and engineering approaches for therapeutic applications
Recombinant technologies have completely changed how we approach therapeutic antibody production. Cells adapted to these strategies are adapted to fast-growth and suspension culture conditions. Moreover, decades of genetic research and manipulation have created the two most important workhorses in antibody production: CHO and HEK293. In this article, we briefly discuss their origin, advantages for recombinant antibody production, current methodologies, and future perspectives.
- Early approaches to antibody production – cells and methodologies
- Chinese hamster ovary (CHO) cells
- Origin, antibody production, and cell derivates
- Typical selection markers in CHO – the DHFR system
- Typical selection markers in CHO – the GS system
- The future of CHO-based antibody production
- Human embryonic kidney (HEK) cells
- Origin, antibody production, and cell derivates
- Typical selection markers in HEK293
- The future of HEK-based antibody production
- Concluding remarks
Early approaches to antibody production – cells and methodologies
Early approaches to the production of monoclonal antibodies relied on hybridoma cell lines. However, these hybrid cell lines were characterized by fluctuating production yields and frequently lost the antibody-encoding genes during long-term cultivation. These limitations have made early antibody production expensive and hindered its translation to large-scale therapeutic production.
However, these constraints started dissolving with the advent of recombinant technologies. These allowed researchers to clone antibody-encoding genes into different expression hosts and to engineer these hosts for large-scale and long-term production.
From an industrial point-of-view, these expression hosts needed to possess certain characteristics. For instance, they needed to be able to perform correct protein folding and adequate post-translational modifications. Moreover, they should also guarantee product safety for human and animal use (i.e. free from contamination with pathogenic agents). Ideally, these systems should also adapt to growth in suspension, as these conditions can be easily scalable for usage in large stirred-tank bioreactors, unlike the lab-scale practices of cultivating cells in adhesion cultures.
Presently, recombinant technologies and genetic engineering have shaped the landscape of antibody production. Cells used for these purposes are mostly from mammalian origin. This marked preference towards mammalian cells stirs from the fact that they have a proven track record for producing glycoproteins with established therapeutic efficiency.
Interestingly, transfected mammalian cell lines are also prone to secreting the proteins to the medium, allowing researchers to obtain high production yields with reduced purification costs.
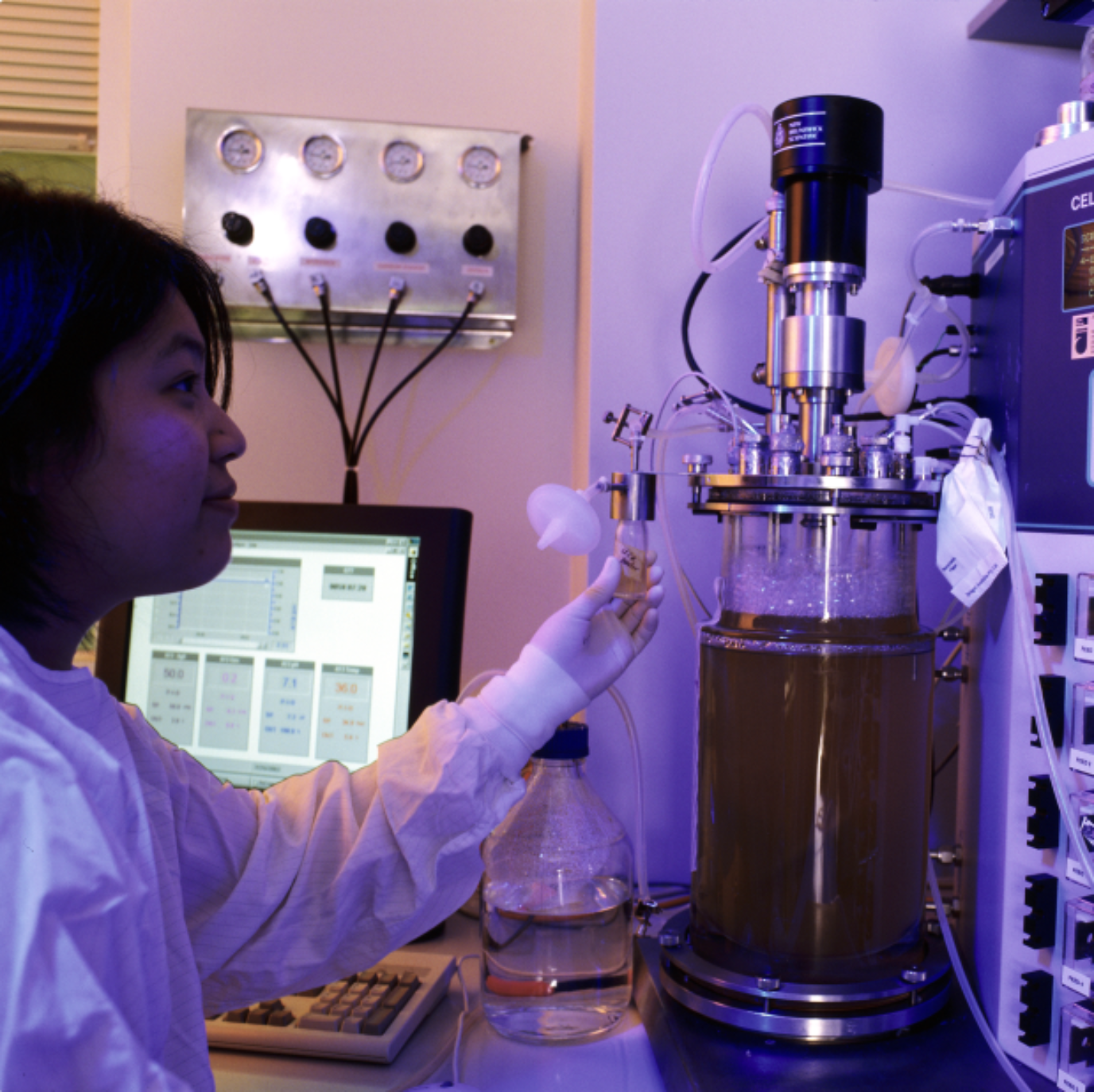
In today’s landscape, the most widely used cell lines are:
- Chinese hamster ovary (CHO) cells
- Human embryonic kidney (HEK) cells
- Baby hamster kidney (BKH) cells
- Mouse myeloma (NS0)
- Per.C6 cells
In this article, we will focus on the first two cell lines, that have become today’s industry workhorses for the production of therapeutic recombinant proteins.
Chinese hamster ovary (CHO) cells
Origin, antibody production, and cell derivates
CHO cells are today industry’s most important workhorse for therapeutic protein production. Chinese hamsters were first used as laboratory specimens as early as 1919, however, cell cultures were only obtained in the late 1950s by Theodore Puck (University of Colorado, USA). These cultures containing cells isolated from an ovary of a female Chinese hamster soon revealed to be resilient, fast-growing and quick to adapt to in vitro cultivation conditions.
Interestingly, the ease of genetic manipulation by mutagenesis has led to the establishment of many CHO-derived daughter cell lines with improved properties. Today, the most important CHO-derived cell lines include CHO-K1, CHO-S, CHO-DXB11, and CHO-DG44.
CHO’s main variants were mostly obtained by random mutagenesis and selection of the original parental cell line. For instance, CHO-S was obtained during the efforts of adapting CHO to the growth in suspension cultures, essential for the large-scale production of biopharmaceuticals. While K1 was cloned directly from the original cell line and later found to lack a gene necessary for glycine synthesis, a deficiency that paved the way for the creation of mutants with high value for recombinant transfection and selection.
Typical selection markers in CHO – the DHFR system
There are currently two main systems for the selection of transfected CHO clones. The first system was established with the CHO-DXB11 variant, obtained by chemical mutagenesis with ethyl methanesulfonate of a CHO cell line. The random mutagenesis which caused the alteration of the two alleles of the dihydrofolate reductase gene (dhfr). Hence, the DHFR- mutant could not synthesize several molecules including glycine, thymine, and purine.
This ability could be easily restored by transfecting the DXB11 with a functional copy of the dhfr. This rendered the cells susceptible to selection by co-transfecting the antibody-encoding genes with a copy of the selective element – the dhfr gene. In this case, only transfected cells would be able to grow in medium without glycine, hypoxanthine, and thymidine, three essential factors for nucleotide synthesis in the DHFR- mutants.
However, soon researchers found that DXB11 cells had limited usefulness, as they could spontaneously revert to a functional dhfr gene, and thus eliminating the possibility to recover positive transfected clones by culturing in selective medium.
This drawback led to the generation of the CHO-DG44 cell line. Unlike DXB11, DG44 mutants were obtained by mutagenesis with gamma radiation and this process caused the irreversible deletion of both alleles of the dhfr gene. For this reason, to this day DG44, it is still one of the dominant CHO cell lines on the market.
The robustness of DHFR selection was further improved by coupling this system with cultivation under high levels of methotrexate (MTX). Methotrexate is a competitive analog of folic acid, the natural substrate of the DHFR enzyme. Hence, its presence induces the amplification of the dhfr gene linked to the antibody-encoding genes and, thus, it has been recurrently used to generate clones with several copies of these genes and, consequently, with high productivity levels.
However, this additional step of progressive selection has also considerably increased the timeline for cell line generation.
Typical selection markers in CHO – the GS system
This limitation has motivated the development of additional selection systems. The most relevant of these is the glutamine synthetase (GS) selection system. This system makes use of the activity of GS, which catalysis the condensation of glutamate and ammonia to generate glutamine.
GS is the only enzyme able to perform de novo glutamine synthesis, moreover, since it uses ammonia for the process, it also prevents tissues from accumulating toxic levels of this metabolite. The GS gene is a dominant selectable marker, without glutamine in the growth medium or GS activity, cells cannot survive. The system was initially designed for cell lines that don’t express sufficient amounts of the GS enzyme such as mouse myeloma (NS0), but it was successfully adapted to CHO cell lines with basal GS expression by adding to the medium MTX, which is also a powerful GS inhibitor. In this case, positive transfection can be selected by culturing in medium containing both MTX and glutamine.
Compared to the DHFR/MTX system, the GS-based selection has a short turnaround time and, in principle, doesn’t require GS knockout. However, GS-based selection also suffers from a relatively lower selection efficiency in comparison to the DHFR/MTX. In fact, some non-transfected CHO cells can survive in extremely high concentrations of MSX (5 mM), which increases the frequency of non-transfected mutants recovered during the selection step.
The future of CHO-based antibody production
The first approved therapeutic monoclonal antibody produced in a CHO cell line was Rituxan, an anti-CD20 antibody used for the treatment of non-Hodgkin’s lymphoma (Genentech, 1997). But since then, researchers have generated impressive amounts of data on CHO genetics, which recently culminated with the determination of the CHO genome in the early 2010s. This discovery will certainly lead to a new generation of engineered CHO variants optimized for selection and gene amplification.
Human embryonic kidney (HEK) cells
Origin, antibody production, and cell derivates
As the name indicates, HEK cell lines were originally isolated from human embryonic kidney cells grown in tissue culture. The cell line we popularized by scientists from the University of Leiden (The Netherlands), who transfected normal HEK cells with the DNA of sheared adenovirus 5. This transfection resulted in the insertion of approximately 4.5 kb of the viral genome into chromosome 19. The clonal subpopulation became known as HEK293 because it resulted from the 293rd attempt at transfecting the parental HEK cells.
The incorporation of this region of the viral genome conferred HEK cells the ability to recognize the CMV promoter region and, thus, it can be used to increase expression levels of vectors harboring the CMV promoter region.
The HEK293 variant was further used as the basis of subsequent transfection experiments. The two most relevant variants that resulted from these experiments are variant HEK293T and HEK293E. The former resulted from transfection with the large antigen T of simian virus 40 (SV40). While the latter resulted from transfection with the nuclear antigen 1 (EBNA1) of the Epstein Barr virus (EBV).
These transfected variants can recognize the origin of replication (ori) of the SV40 and EBV, respectively. Hence, they are capable of carrying out the semi-stable episomal propagation of vectors containing these ori.
HEK293-derived cell lines are thus easily transfected and can grow quite rapidly in suspension cultures. However, the main drawback of therapeutic antibody production using these cells is their origin. Since HEK are of human origin, there is a risk of contamination with human-specific viruses.
Typical selection markers in HEK293
Unlike CHO-derived cell lines, which make use of metabolic selection markers, HEK293-based production usually makes use of antibiotics to select positive transfected clones. For instance, for antibody production, cells are traditionally transfected with one of the following systems:
- Blasticidin – this antibiotic inhibits the termination of protein translation, and resistance to this drug can be conferred by the bsd gene
- G418/Geneticin – this antibiotic inhibits peptide chain elongation and resistance can be conferred by the neo gene
- Hygromycin B – like G418, this antibiotic inhibits chain elongation and resistance can be conferred by the hygB gene
- Puromycin – inhibits protein synthesis, resistance can be conferred by the pac gene
- Zeocin – it complexes with the DNA causing strand scissions, resistance can be conferred by transfection with Sh bla gene
The future of HEK-based antibody production
Given the high productivity and ease of transfection, many researchers have been looking for alternatives to antibiotic-based selection in HEK293 and corresponding derivates. Recently, researchers from the Autonomous University of Barcelona explored HEK293’s natural and genetically engineered auxotrophies.
These researchers tested different approaches:
- Culturing in tyrosine-free medium and exploring the natural tyrosine auxotrophy of HEK293 cells and derivates. Positive transfected clones can be selected by including a pah gene (phenylalanine hydroxylase) in the expression vector
- CRISPR/Cas9 knockouts defective in thymidylate synthase (TYMS)
- CRISPR/Cas9 knockouts defective in glutamine synthetase (GS)
They found that the PAH system proved to be a highly efficient selectable marker when compared to well-established commercial systems. Moreover, GS-knockouts also showed promising results. Nevertheless, more research regarding culture medium optimization is necessary to make these systems compatible with industrial use.
Concluding remarks
Over the years, many methodologies have been established for antibody production. Cells used for these purposes are often from a mammalian origin, due to their natural ability to perform correct protein folding and the desired post-translation modifications, which are vital to guarantee the efficiency and safety of therapeutic antibodies.
Presently, CHO and HEK293-based production are the dominant technologies for antibody production. These cell lines can be easily adapted to grow in suspension cultures. Moreover, decades of research have rendered them amenable to selection using well-established metabolic or antibiotic resistance markers. Due to increasing knowledge regarding the genetics of these cell lines, researchers will probably keep using these parental cell lines as the basis for the generation of better antibody-producing systems.
- Dangi, A. K. Cell Line Techniques and Gene Editing Tools for Antibody Production: A Review. Front Pharmacol. 2018; 9: 630. doi: 10.3389/fphar.2018.00630
- Fan, L. et al. Improving the efficiency of CHO cell line generation using glutamine synthetase gene knockout cells. Biotechnol Bioeng. 2012; 109(4):1007-1015. doi: 10.1002/bit.24365
- Frenzel, A. et al. Expression of Recombinant Antibodies. Front Immunol. 2013; 4: 217. doi: 10.3389/fimmu.2013.00217
- Graham, F. L. et al. Characteristics of a human cell line transformed by DNA from human adenovirus type 5. J Gen Virol. 1977; 36(1):59-74. doi: 10.1099/0022-1317-36-1-59
- Jayapal, K. P. Recombinant Protein Therapeutics from CHO Cells – 20 Years and Counting. Chem. Eng. Prog. 2007; 103: 40-47.
- Reinhart, D. et al. Bioprocessing of Recombinant CHO-K1, CHO-DG44, and CHO-S: CHO Expression Hosts Favor Either mAb Production or Biomass Synthesis. Biotechnol J. 2019; 14(3): e1700686. doi: 10.1002/biot.201700686
- Roman, R. et al. Enabling HEK293 cells for antibiotic-free media bioprocessing through CRISPR/Cas9 gene editing. Biochem Eng J. 2019; 151: 107299. doi: 10.1016/j.bej.2019.107299
- Urlaub, G. et al. Deletion of the diploid dihydrofolate reductase locus from cultured mammalian cells. 1983; 33(2):405-412. doi: 10.1016/0092-8674(83)90422-1