A guide to mammalian cell-based expression systems
Mammalian cell-based expression systems are the dominant technology used in the production of biopharmaceuticals. These expression systems excel in terms of protein folding and post-translational modifications, often crucial for the activity and therapeutic effectiveness of recombinant proteins. To help you understand if these expression systems are the answer to your needs, we have compiled key information regarding the advantages and principles of mammalian expression systems.
- When to choose mammalian cell-based systems to express recombinant proteins?
- How to choose between transient and stable expression?
- XtenCHO™ Expression System
- XtenCHO™ – transient expression with ultra-high yields and minimal hands-on time
- XtenCHO™ – the ideal expression system for biopharmaceutical screening applications
- Applications of recombinant proteins produced XtenCHO™
- Principles of transient protein expression in mammalian systems
- Cell lines used for transient expression
- Vector design for transient protein expression
- Transfection of mammalian cells
- Protein expression and purification
When to choose mammalian cell-based systems to express recombinant proteins?
Mammalian cell-based expression systems are optimal for the production of large proteins with complex native structures and activity. In comparison to lower eukaryotes or bacterial systems, mammalian cells excel at:
- Protein folding
- Post-translational modifications (human-like)
- Provide ultra-high protein yields
- Possess highly efficient protein secretion pathways resulting in low-cost protein purification and high purity
- Ensure an enhanced batch-to-batch consistency meeting important quality standards for therapeutic protein production
- Allow diversified methodology (transient versus stable) and cell lines
Due to these properties, the vast majority of therapeutic proteins on the market are currently produced in high-yield mammalian cell lines such as Chinese Hamster Ovary (CHO) cells. These cells are adapted to growth in chemically defined and animal-free media. Moreover, they support high-density culture conditions which help to maximize production yields while keeping culture volumes low.
Beyond the clinic, many proteins are produced in mammalian systems. The main non-therapeutic applications include structural and functional studies of mammalian or human proteins – vital for clinical and fundamental research. These systems may also be used to produce enzymes or other functional proteins for analytical and research applications. Moreover, mammalian cells can be used to produce non-mammalian recombinant proteins when these molecules fail to be produced in other expression systems.
How to choose between transient and stable expression?
Mammalian cell-based systems can either express a protein in a transient or stable way. Both approaches have their advantages and limitations. In this way, they cater to different applications or can be used at different stages of the protein development process.
Unlike other expression systems, mammalian cells are unable to recognize plasmid origins of replication and, thus, cannot replicate DNA vectors independently from their genome. This limitation prompted the development of transient and stable systems. The first type of system leverages the long cell cycles of mammalian organisms to allow the expression of recombinant proteins for limited periods (from 5 to 14 days). These systems are typically transfected with high copy numbers of the expression vector, which are eventually lost after a few cell division cycles. Despite the transient nature of these systems, one of their greatest advantages is their short lead times and high yields. These features make them invaluable for applications such as drug and protein variant screening or research (basic or medical).
In contrast, stable expression is a time-consuming process requiring the long-lasting integration of the DNA vector into the genome of the mammalian system. This integration can be random or site-directed (mediated by transposons) and it requires the selection and isolation of single positive clones after transfection. Single-clone screening is the most time-consuming step of the process, usually requiring up to 4 months for completion. It is routinely achieved by manual methods such as limiting dilution or automatized procedures such as VIPSTM (verified in-situ plate seeding). The long periods required to generate stable cell lines have made this method useful for biopharmaceutical production at a large scale.
XtenCHO™ Expression System
XtenCHO™ – transient expression with ultra-high yields and minimal hands-on time
The XtenCHO™ Expression System maximizes protein yield, extends transient expression periods, and minimizes hands-on time. Based on a high-yield Chinese Hamster Ovary (CHO) cell line, this transient system was designed to deliver up 10x more protein in comparison to conventional CHO-S systems, while enhancing protein folding and performing essential human-like post-translational modifications.
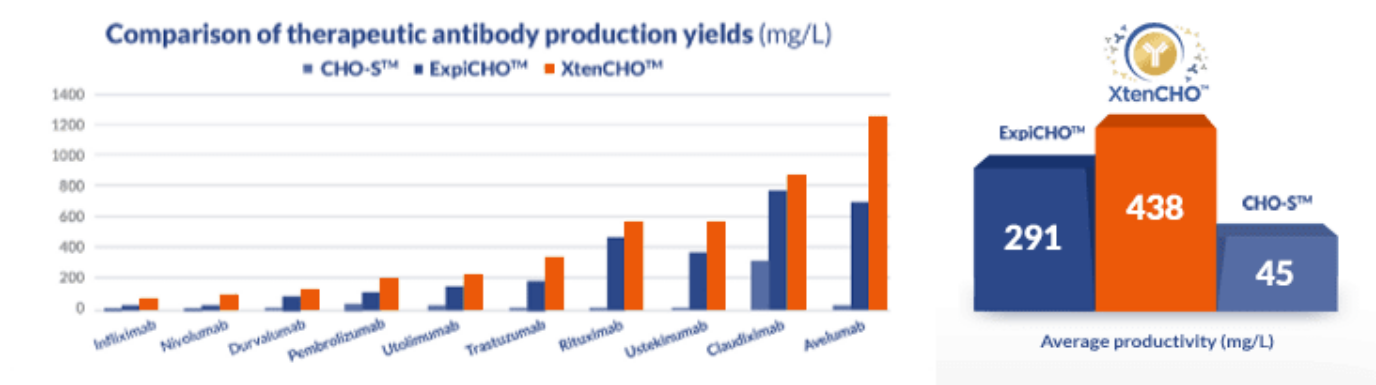
Get the most out of your transient expression assays
- Up to 10x more protein without supplemental feeds
- 3x longer transient expression periods
- Minimized hands-on time thanks to the one-step transfection protocol
- Smart protocols for easy integration into your in-house projects and platforms
Hassle-free and rapid protein development
• Enhanced protein folding and post-translational modifications ideal for clinical research and drug screening applications
• Flexible purchase options adapted to your needs. Rapid shipping (in 24h or less). Worldwide delivery in 3-5 business days.
XtenCHO™ – the ideal expression system for biopharmaceutical screening applications
Almost 95% of protein drug candidates fail to reach the market or clinic due to undetected developability issues. Experts agree that this high failure rate can be mitigated by performing lead screening during early development and thorough characterization as soon as the best leads are selected for further development. The XtenCHO™ transient expression system allows the rapid production of up to 1.28 g/L of recombinant protein with glycosylation profiles and structure comparable to those achieved by stable cell lines (stable CHO-K1) and other commercial systems.
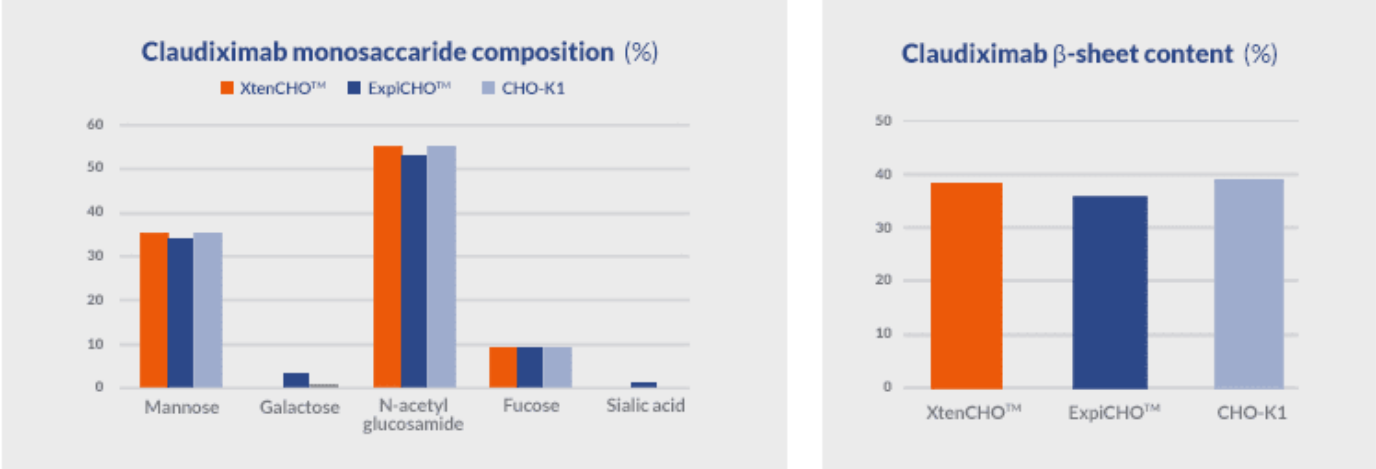
The strong correlation in terms of structure and post-translational modifications makes XtenCHO™ an ideal expression system for the evaluation of early drug candidates and downstream characterization in terms of:
- Specificity and selectivity – specificity indicates the drug’s ability to bind to its specific target, while selectivity indicates if it binds to a single target or multiple receptors. A drug can be specific but not selective. In this way, these properties must be tested in vitro before further development.
- Affinity and avidity – these two properties are used to characterize the binding strength between a ligand (antibodies or other drugs) and its target. The term affinity is used to describe the strength of the interaction at the binding site formed by a combination of hydrogen bonds, hydrophobic interactions, electrostatic and van der Waals forces. In contrast, avidity measures the overall strength of the ligand-target complex determined by the affinity, valency (total number of binding sites), and the structural arrangement of the complex.
- Immunogenicity – highly immunogenic drugs are prone to causing adverse reactions in patients and to be more quickly cleared from the organism. In this way, developing drug with low immunogenicity is key to ensuring the safety and longevity of new treatments.
- Solubility – highly insoluble drugs are prone to forming aggregates leading to limited therapeutic effectiveness.
- Stability – drugs that are more susceptible to proteolytic degradation have a limited shelf life. In this way, testing drug candidates for degradation early on allows engineering protein structures in a way that shields these sites, making them less accessible to proteolytic enzymes.
- Ease of purification – transient production allows the estimation not only of production yields but also of ease of purification, allowing the optimization of affinity purification tags at a small scale.

Get more out of your transient expression assays
Discover how XtenCHO™ can boost recombinant expressionApplications of recombinant proteins produced XtenCHO™
Drug candidate screening | Protein variant screening | Analytical and research applications |
The XtenCHO™ system is comparable to stable CHO-based systems used for large-scale biopharmaceutical production. With outstanding ability to perform superior protein folding and post-translational modifications, this system makes it easier to evaluate your drug candidates during early development in a cost-effective way. | Protein engineering by random or site-directed mutagenesis tends to generate multiple variants that require validation before further development. The smart protocols and rapid generation times of XtenCHO™ allow the use of this system to quickly and robustly screen variants obtained from antibody humanization, in vitro affinity maturation, stability improvement, among other protein engineering processes. | Achieve ultra-high titers without sacrificing quality, purity, or protein activity ensures that you can easily integrate XtenCHO™ production into your everyday workflows. By getting highly pure, easy to purify stocks in a short amount of time, you will save costs and time in your research applications (immunoassays, analytical processes, among others). |

Get more out of your transient expression assays
Discover how XtenCHO™ can boost recombinant expressionPrinciples of transient protein expression in mammalian systems
Transient expression in mammalian systems allows the rapid production of recombinant proteins with complex structures, activity, or large size. These systems are ideal for routine expression for diverse applications. Two of their most important advantages are the flexibility in terms of protein quantity and the availability of fully optimized systems for seamless integration into protein development platforms or projects.
The production of recombinant proteins in these systems starts by selecting the target protein; designing an expression vector with the adequate promoter, selectable markers, and expression tags (optional); cloning the gene of interest (codon-optimized) into the vector; transfecting the selected host; finally producing and purifying the recombinant protein.
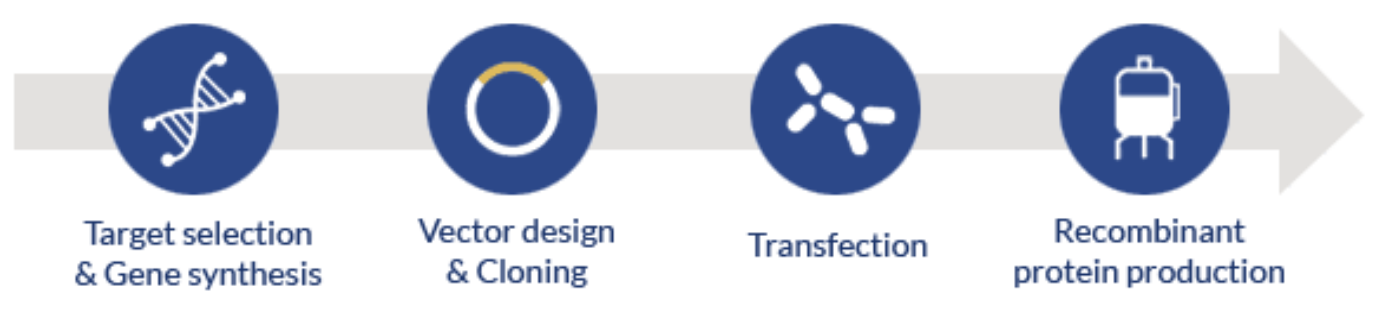
Due to their limited capacity for plasmid retention, transient expression hosts are rarely subjected to clonal selection. Instead, these systems are designed to maximize transfection efficiency because the number of plasmids successfully inserted in mammalian cells generally correlates with the final production yield. In this way, when optimizing cell lines and reagents for transient expression, researchers often use reporter systems to measure transfection efficiencies rather quickly. A few examples and methodologies can be found below:
- Green Fluorescent Protein (GFP) – discovered in marine organisms such as jellyfish, GFP has since become one of the most widely used reporter systems. Cells expressing GFP can be directly visualized under a fluorescence microscope. However, for the quantitative determination of transfection efficiency, the use of high-throughput and highly sensitive methods such as flow cytometry is recommended.
- Luciferase – originally found in fireflies and click beetles, luciferase produces bioluminescence by catalyzing the oxidation of luciferin in an ATP-dependent reaction. The co-transfection of this gene together with the gene of interest allows the straightforward quantitative readout of photon emission that helps to determine relative transfection efficiency between samples.
- β-galactosidase – encoded by the lacZ gene in Escherichia coli, β-galactosidase catalyzes the hydrolysis of β-galactosides such as o-nitrophenyl-β-D-galactopyranoside (ONPG). The hydrolysis of ONPG in cell lysates of transfected cells converts the colorless substrate into galactose and the chromophore o-nitrophenol, resulting in a bright yellow solution. Because this chromophore emits on the visible spectra, β-galactosidase assays are inexpensive and can be carried out on a simple spectrophotometer or microplate reader. The sole disadvantages of this assay are the need to lyse cells for quantification and the presence of endogenous β-galactosidase in some cell types.
Cell lines used for transient expression
The industry’s dominant workhorses for transient expression are Chinese Hamster Ovary (CHO) and Human Embryonic Kidney (HEK) cell lines. Noticeably, in the production of biopharmaceuticals, CHO cells continue to be the preferred host. Currently, about 70% of all FDA-approved biopharmaceuticals are produced in CHO cells, while HEK-produced therapeutic proteins have yet to reach the market.
Although HEK cells are of human origin and, thus, able to perform fully human post-translational modifications, handling these cells represents a moderate health biohazard since they can carry and transmit human pathogens. In contrast, CHO cells are considered a safer alternative with comparable and human-like glycosylation profiles. Thus, explaining the preference towards these hosts in the large-scale production of biopharmaceuticals.
Interestingly, initial efforts to use CHO cells for transient production found limited success due to low transfection efficiencies. This led to the quick development of HEK-based transient systems that, conventionally, have higher transfection rates. However, data shows that the early and preclinical development of new biopharmaceuticals in HEK cell lines and later development in CHO cell lines increases the risk of late-stage development failures due to the differences in terms of structure and biophysical properties achieved by each of these hosts.
In this way, when a new protein is intended for therapeutical development, CHO-based transient and stable production should be preferred. Presently, the development of new transfection technologies resulted in a significant enhancement of transfection rates in CHO cell lines leading to the proliferation of highly productive CHO-based transient systems.
Vector design for transient protein expression
To maximize production yields, genes of interest are typically subjected to codon optimization to enhance transcription in the expression host. The codon-optimized product is subsequently cloned into a suitable and high-yield expression vector.
A typical expression vector used in mammalian systems includes the following elements:
Element | Description | Examples |
---|---|---|
Gene of interest | The gene that encodes the target protein. | Antibody, therapeutic protein, etc. |
Promoter | Mammalian systems typically employ strong constitutive promoters to achieve high levels of expression without the need to use additional signals such as heat or chemicals – typically required when inducible promoters are used. | CMV (human cytomegalovirus); SV40 (simian vacuolation virus 40); EF1A (human elongation factor alpha). |
Selectable marker | Metabolic selection is typically employed in CHO cell lines while antibiotic selection is the preferred approach for the selection of HEK cells. | DHFR (dihydrofolate reductase) and GS (glutamine synthetase) and the dominant metabolic selection systems. Antibiotics such as blasticidin, puromycin, G418/geneticin, hygromycin B, and zeocin may be used for mammalian cell-based selection. |
Reporter gene | These genes may be included in initial experiments to determine transfection efficiencies. Alternatively, they can be used in functional studies to localize the expression of particular genes within the cells. | GFP, YFP (yellow fluorescent protein), RFP (red fluorescent protein), luciferase, β-galactosidase, and mCherry, among others. |
Protein tag | Protein tags can be classified according to their purpose. Affinity or epitope tags are used to improve purification efficiencies and solubility tags to improve solubility. Some solubility tags also facilitate the affinity purification of recombinant proteins. | Purification: HA (hemagglutinin), Poly-His (polyhistidine), Strep-tag (streptavidin), CBP (calmodulin-binding protein).
Solubility: MBP (maltose-binding protein), GST (glutathione-S-transferase), SUMO (small ubiquitin-like modifier). |
Others | Signal peptides can be used to improve translocate the protein of interest to the cell membrane and enhance protein secretion; protease recognition sites can be used to allow the cleavage of the peptide link between tags and the protein of interest; among others. |
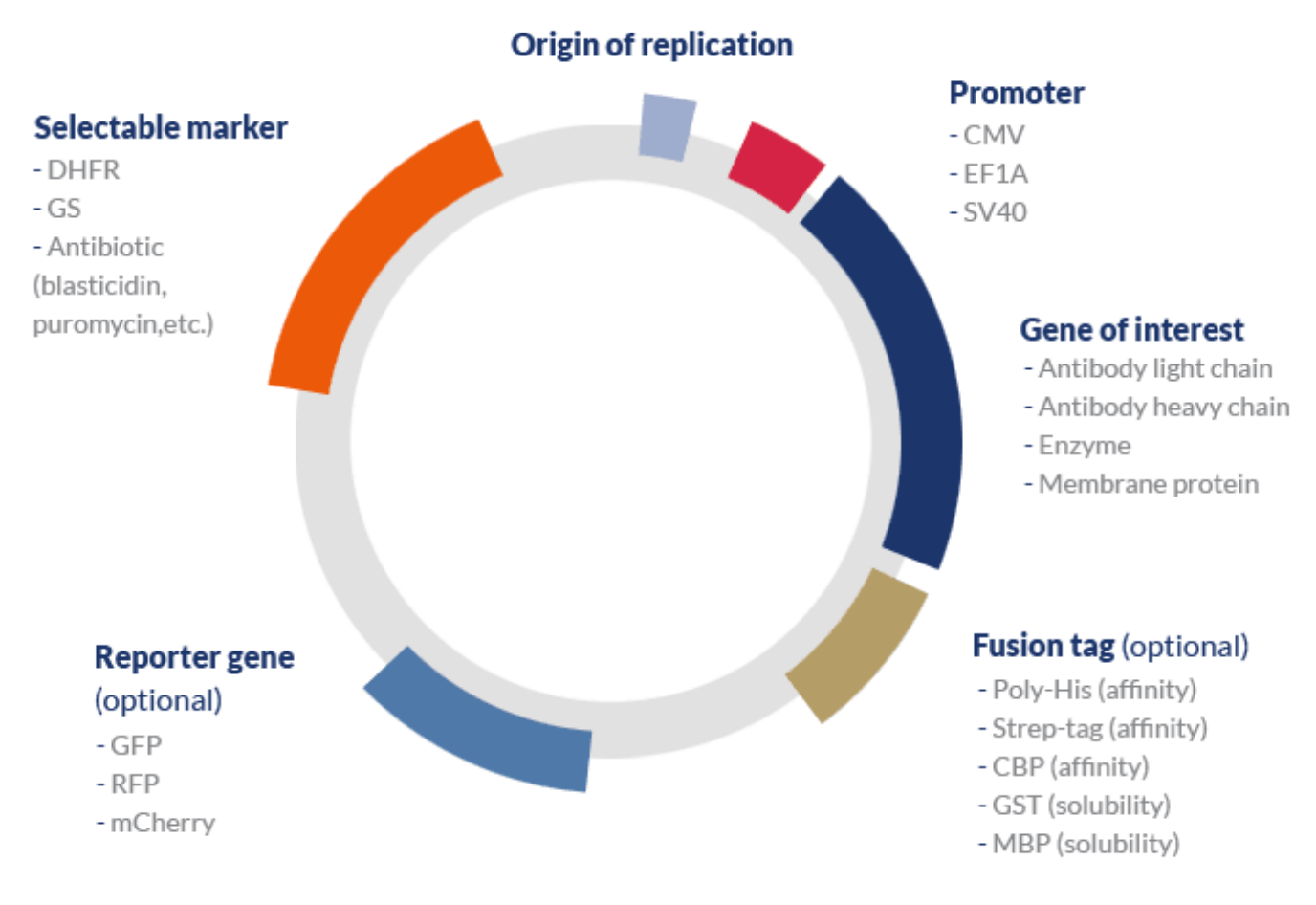
Transfection of mammalian cells
Mammalian cell-based systems generally employ plasmids (transfection) or viruses (transduction) for the introduction of expression vectors into the host. Although transduction methods show high efficiency, viral particles need to be propagated in cells (often mammalian) significantly extending lead times and increasing production costs.
For this reason, transient mammalian systems typically employ plasmids in which the gene of interest has been inserted (cloning). After cloning, the mixture of plasmids with or without inserts is propagated in Escherichia coli for the selection of correctly assembled plasmids and their subsequent amplification. Plasmids produced via amplification in E. coli need to be thoroughly purified and subjected to endotoxin removal – a toxic by-product found in E. coli growth medium that is toxic for mammalian cells. Alternatively, plasmids containing the gene or genes of interest may be fully synthesized in vitro resulting in higher purity and enhanced transfection efficiency.
The transfection of mammalian cells for transient protein expression is currently achieved using the following methods:
- Chemical methods – the use of chemicals to introduce highly negatively charged nucleic acids through the phospholipidic cell membrane is the most widely used approach for mammalian cell transfection. In general, these methods are more cost-effective, less labor-intensive, and less prone to affect the viability of cells. Some of the most commonly used chemical transfection methods include:
- Calcium phosphate – this method is based on the formation of calcium phosphate-DNA precipitates. The calcium allows the complex to bind to the cell membrane. As a result, the DNA is uptake by the cells by endocytosis.
- Lipotransfections – liposomes act as analogs of the phospholipid bilayer. in this way, by encapsulating free DNA, they allow the efficient uptake of the complex by endocytosis.
- Cationic lipid transfection – this method results from the fusion of liposomes with cationic polymers, which tend to form strong complexes with negatively charged nucleic acids. These complexes are also absorbed by the cells by endocytosis and are currently considered one the most effective chemical methods.
- Physical methods – in contrast to chemical methods, physical methods imply the use of a physical force to introduce plasmid vectors into cells. These methods are generally considered more effective than chemical methods; however, they also tend to disrupt the viability of cells. Thus, they require a higher number of cells and a more laborious process of optimization. The most commonly used physical method of transfection is:
- Electroporation – this method uses electrical pulses to create temporary pores in cell membranes. By doing so, it makes membranes more permeable to nucleic acids which are then free to enter the cytoplasm without the need for further adjuvants.
Protein expression and purification
Most recombinant proteins are fused with affinity (protein) or epitope (peptide) tags. These tags allow the rapid purification from the culture medium either by immobilizing compounds or target-specific antibodies on resins (affinity chromatography) or magnetic beads (magnetic-based purification). Affinity chromatography tends to be the method of choice for large-scale production, while magnetic beads, although highly efficient, can become expensive at a large scale. For this reason, magnetic-based purification is typically reserved for small and medium-scale production.
Recombinant antibodies are alternatively purified using the following methods:
- Immunoglobulin-specific purification: a wide range of microbial proteins is known to bind strongly to antibody’s Fc or Fab fragments. A few examples are protein A (Staphylococcus aureus), protein G (Streptococcus), fusion protein A/G, and protein L (Peptostroptococcus magnus). These proteins can be immobilized into resins and easily used to capture antibodies from the culture medium.
- Antigen-specific purification: alternatively, antibodies can be captured by immobilizing their specific antigen in a resin.

Whether you are working with hard-to-express proteins or aiming to optimize your production yields, our solutions are designed to meet your every need.
Discover our custom protein production platform